Heart disease accounts for almost half of all deaths in the developed world. The most effective treatment is still heart transplants, but the demand for donor organs far exceeds supply. To address this imbalance, engineers at the University of Leeds have embarked on a project that could transform the way cardiovascular illnesses are managed.
The academics, from the school of mechanical engineering, have developed an intelligent device that could be wrapped around a damaged heart, applying compression to boost blood flow. They have also built a mechanical heart simulator so they can test the device over long periods to measure its performance.
“It’s an exciting development,” says Dr David Keeling, the lead engineer on the project. “The heart-assist device effectively acts as an artificial muscle wrap, surrounding the ventricles and squeezing in synchronisation with the heart’s native rhythm.
“We have also developed what we believe is a realistic, reliable, and reconfigurable testing environment to advance and improve the device without the need for animal testing.”
The intelligent ventricular-assist device (iVAD) has been designed to function as an artificial muscle wrap that assists the failing heart by applying compressive force around the external surface of the ventricles. This cyclic “squeezing” action augments heart muscle efforts, improving output by as much as 25%.
Keeling and his colleagues needed to physically apply the iVAD to a heart simulator to measure its compressive efforts, so a realistic in vitro testing environment was imperative for development. Approaches used for other heart-assist devices have involved either mechanical mock circulatory systems or extracted hearts metabolically supported by another animal’s blood flow.
“Traditional methods use excised hearts or bulky hydraulically or pneumatically driven systems – we preferred neither method,” says Keeling. “We wanted to come up with a ‘mechatronic’ version. So we created a unique hardware-in-the-loop (HIL) heart simulator that combines a real-time software blood-flow model with a physical 3D mechanical heart.
“The hardware is a mechanical heart that feeds into a simulated loop which has a numerical model of blood flow throughout the body. The heart rhythm simulation is then mimicked by the mechanical heart.
“We can then put the assist device onto the mechanical heart to squeeze it, allowing us to measure the compressive pressure. That pressure gets fed into a simulation, and from that we can work out how effectively the device would assist the patient.”
The researchers wanted the heart simulator to be reconfigurable so that it could physically and haemodynamically replicate different patient groups, illnesses, and
animal models. This adaptability could reduce the need for animal testing, because the heart simulator could be used for prolonged trials of prototype iVADs and provide information on physiological effects.
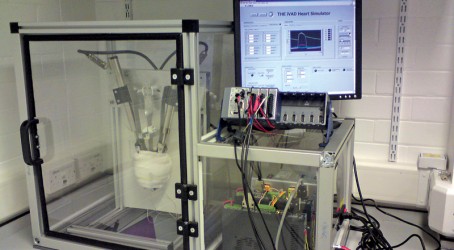
With an assist device such as the iVAD, the interaction between it and the heart surface is crucial. This is likely to depend on physical features that are difficult to model, such as backlash and nonlinear friction. Therefore, it was vital that the heart simulator had a physical object onto which the researchers could apply the iVAD and monitor its raw compressive operation.
The researchers based the heart simulator design on HIL simulation, which simulates components from a system with software and links these to specified physical hardware parts from the same system that require testing. To satisfy the heart simulator requirements, they used HIL simulation for a mechanical heart functioning as hardware within a simulated blood-flow model loop. The continuous feedback loop between the two is used to assess how the device’s physical assistance affects the heart and blood flow if implanted inside the body.
“The mechanical heart’s shape is defined by two modifiable semicircular patterns of buckled spring steel strips, which are attached at both ends with adjustable boundary conditions,” says Keeling.
“We developed a custom-built National Instruments vision program to help define the necessary boundary conditions to match the profile of each steel strip to a reference heart model. We used two linear actuators to cyclically flex the steel strips and realistically represent the dynamic movement of the heart’s right and left ventricles.
“We control the actuator’s motion within the blood-flow model to mimic the simulated heart, so any volume change to the simulated heart is immediately reflected by the physical heart. In addition to matching the heart shape, the arrangement potentially varies local stiffness around the circumference of the mechanical heart by individually altering the mechanical properties of the strips, such as thickness. We use a thin elastic skin to surround the strips and apply the iVAD.”
In terms of the feedback loop to assess the iVAD assistance to the cardiovascular system, four conformable pressure sensors were located at equal intervals around the mechanical heart to provide iVAD assistance (compression) data. Within the model, this data was converted to an assistive pressure for each ventricle, and the subsequent effect on the blood flow was calculated in real time and caused associated changes in the motion of the mechanical heart.
The blood-flow model operates as a closed-loop lumped parameter model based on an electrical network analogy. Because each region of the heart is modelled separately, the researchers could control the heart locally and implement specific heart conditions and diseases.
“To help satisfy one of our main goals, the blood-flow model can be automatically fitted to represent real physiological data through the use of a nonlinear least-squares parameter estimation method, implemented as a state within the National Instruments LabView code,” says Keeling. “This means the heart simulator can accurately reflect the haemodynamics of most patient groups and in vivo models, helping to improve our understanding of the device’s potential effect.”
The work is ongoing, although stringent medical regulations mean the insertion of an iVAD into a real-life patient is still a long way off. But Keeling reckons that the device has the potential to boost cardiac output by up to 25%. “There would need to be in vivotests to validate this,” he says. “And the project requires more research into material suitability. But we think the work has real potential.”