Diagnosing disease can be a messy business. Patients can be subjected to biopsies, endless blood tests and other “explorations” as doctors try to figure out what is wrong or monitor symptoms. But researchers in the field of medical optics are trying to change all this. They are working to develop tests that can monitor wellbeing, and even diagnose cancer, simply by shining light on tissues. Crucially, these techniques do not require tissue samples cut with a surgeon’s scalpel, or blood drawn with a nurse’s syringe.
One group using these techniques is the biomedical engineering research team at City University London, led by Professor Panicos Kyriacou. They have developed a series of devices that use the principles of optics to monitor the health of patients undergoing surgery, or recovering from it, in precise ways that have not been possible before.
During surgery, doctors need to check how well oxygen is flowing around the body. They usually do this using a pulse oximeter – a small device that clips onto the end of a patient’s finger, toe or ear lobe and measures the oxygen being carried in the blood by haemoglobin.
The trouble with these devices is that they can measure blood oxygenation only in the area where they are fixed. When patients are very ill or undergoing major surgery, the body begins to shut down the arteries that supply blood to outer areas such as fingers and toes, to help preserve the blood supply to the vital organs. Once these peripheral arteries shut down, a standard pulse oximeter has insufficient quantities of blood to work with, and can give incorrect readings or none at all.
In these situations, the medical team will measure blood oxygenation levels by drawing blood from an artery every 15 minutes and running the sample through a special laboratory machine to perform a blood gas analysis. This machine may not be close to the operating theatre, and the method does not give the continuous monitoring that is often needed in intensive care medicine. And for newborn babies who are premature or very sick, drawing blood can be traumatic – it takes time for their body to settle down again.
Clinicians wanted to get around these problems. So they approached Kyriacou’s team – comprising physicists, engineers, biochemists, materials scientists and software programmers – to see if they could devise a new type of pulse oximeter that could overcome the limits of the traditional design.
Since the mid-1990s, the group have been working on a range of devices that can monitor the oxygen levels of specific parts of the body. The first was a small sensor, on the end of a wire probe, that monitors the oxygenation of blood at the base of the tongue and the start of the oesophagus.
The group first tested the device in adults, and then created a miniaturised version for use with newborn babies.
The sensor proved effective. “We have proven beyond any doubt that when the periphery shuts down, the oesophagus remains continuously perfused with oxygenated blood,” says Kyriacou.
The device works because the colour of blood varies depending on how much oxygen is present. It has two LEDs that emit different wavelengths of light – red and infrared – and a photodiode receptor. Once it is switched on, photons of light scatter around the tissue. Some are reflected and some absorbed – the red light by blood haemoglobin molecules in arteries that are carrying oxygen, and the infrared light by blood haemoglobin molecules that are not.
The photodiode then detects any reflected photons and converts them into an electrical voltage. “Millions of photons are lost in this process; very little light comes back, but it is enough to give us a signal,” says Kyriacou. “In that voltage there is a physiological signal called the photoplethysmographic signal, which has information about both optical wavelengths mixed together. We separate it using multiplexing techniques.”
A ratio is then created that represents the amount of oxygenated arterial blood compared with deoxygenated arterial blood, and the values are plugged into a calibration algorithm to produce a value for the amount of oxygen saturating blood in the arteries.
The light used poses no risk to tissues as it is in the safe spectrum, says Kyriacou. “This is non-ionising radiation, and a very low current.”
Using the same technology, the group have gone on to develop a host of other devices that can help clinicians to monitor patients.
One of these, which has recently completed clinical trials, can be used by plastic surgeons to work out whether tissue taken from the abdomen to reconstruct a breast has successfully grafted with local arteries and veins and has a blood supply. “It is critical, especially on the first day, to make sure that the graft, the free flap, doesn’t fail,” says Kyriacou. “There is no real routine monitor to tell surgeons and nurses that the free flap is doing well.” Instead, surgeons and nurses have had to visually check the site every hour and touch it with the back of their hand to check for warmth – a sign that blood is flowing.
The new device could make this monitoring easier and more effective. “We worked with a team at the Broomfield hospital to develop a reflectance optical sensor that sits on the free flap and monitors any optical signals relating to arterial blood. It might give us a continuous and non-invasive way of measuring the perfusion,” says Kyriacou.
Another of the group’s applications measures perfusion in the brains of newborn babies. It works by shining light through the fontanelle – a soft spot in the skull that exists because the skull bones are not fully fused – to measure brain oxygenation. This technique can help to spot the signs of haemorrhage or hypoxic encephalitis in the infants.
And it’s not just oxygen that the technology detects. Using different colours of light, it can determine the concentration of other molecules in the blood of the brain, too, says Kyriacou. “Many components of blood have a unique absorption of light. Identifying these optical fingerprints enables us to extract the concentration of glucose or carboxyhaemoglobin.”
Most recently, Kyriacou and his team have used the sensors to check the oxygenation levels of specific organs – such as the kidney, bowel, stomach and liver – while a patient’s abdomen is open during surgery at the Royal London Hospital and St Bartholomew’s. “It’s the first time anyone has really brought signals from those parts of the body,” he says.
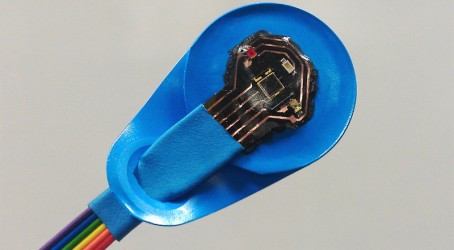
The team have now started to look at using optical technology to study the saturation of oxygen in veins – an application that requires much more signal processing and mathematical computation.
Research into medical optics has been ongoing for years, and there have been many recent developments, according to Michael Neuman, professor of biomedical engineering at Michigan Technological University in the US. For example, Japan’s Masimo Corporation has a version of the pulse oximeter that can determine blood glucose levels, he says. “If it works clinically, and it is easy to use, it would be a great advance.” Currently, diabetes patients measure their blood sugar levels by taking a drop of blood and testing it with a kit.
But for Neuman, the most exciting developments are in applications that combine sensing with imaging. Biomedical engineers at various research sites are looking at the fluorescence of tissues that are easy to access – such as skin, uterine cervix, oesophagus, colon and rectum – under ultraviolet light to help diagnose precancerous lesions. Generally, the optical processing technology sits at one end of a flexible optical fibre that can pass through body cavities such as the gastrointestinal tract.
The operator shines a beam of light into the tissue, and creates an image by looking at the timings of the first reflections. “You can create an image with close to the resolution you can get from light microscopy and staining techniques, showing individual cells and, to some extent, the structure of the cells,” says Neuman. “Its advantage is that you don’t have to cut out the tissue to access it.”
However, getting to the tissue that requires scrutiny can cause problems, as light can penetrate only a small distance. If the precancerous cells pass deeper into the tissue, the light cannot access them. There are also problems with the processing technology that creates an image, which is usually complicated to use and expensive. “A lot of the work is still limited in labs, but there are some commercial devices in ophthalmology and dermatology,” says Neuman.
However, this technology offers great potential if it can be simplified for use at the primary care level, he says. It could help doctors work out whether a patient should be referred to specialist services or not. “Although it is expensive technology, it has the potential to reduce healthcare costs,” he says.
Masimo is not the only organisation to be working on a diabetes device. City University’s biomedical engineering research group is also working to develop sensors for patients with perivascular disease and diabetes, so they can monitor themselves at home. The device would enable them to collect data on their condition remotely rather than having to visit the hospital each week, for example. “This would save money for the country and the NHS, and enable them to deal with more critical cases,” says Kyriacou.
However, getting such sensors tested on humans can prove a hurdle, he says. Few biomedical engineering research groups are testing new devices in patients, because it is getting more difficult to secure access. As a result, many groups are working in the laboratory and with computer simulations and modelling.
“The hoops that you have to jump through to get close to patients – some of them justifiable – are becoming very bureaucratic.” For example, one of the group’s trials, at Great Ormond Street Hospital for Children, took 18 months to get ethical approval.
This preparation time can put biomedical engineers off testing their devices in people, says Kyriacou. “Some people might not be prepared to do that. But there is a lot of value, and the experience is immense. If you develop a medical device and you can technically test that it works only in the laboratory, it will just remain a hypothesis.”
Although his team’s devices have been tested in patients, none is yet available commercially. The group holds four or five patents for the optical devices, and plans to commercialise the technology. How this will be done remains to be seen. The patents could be licensed, or sold as a platform of technologies. The group has not ruled out creating a spin-out company, either. Whichever route they take, the future looks bright for medical optics devices.
Trials show potential for children’s medicine
Professor Andy Petros, consultant paediatric intensivist at Great Ormond Street Hospital for Children, in London, worked with Kyriacou and his team on two of the optics devices: the newborn oesophageal sensor and the fontanelle sensor. As the clinician for the project, he used the devices on patients in practice and is excited by the technology’s potential.
When using the sensors, he saw “fantastic benefits” compared with conventional pulse oximeter devices. “We had one child who was badly burnt, and it was not easy to find a suitable place to put a normal saturation probe. So we were able to put it down the oesophagus and get a good signal,” he says. If the device were to be commercially available, it could become the first-choice option for critically ill children, Petros believes.
The fontanelle monitor could be developed further to glean more clinical information, he says. One option could be in monitoring premature babies for bleeding into the brain. “If you are measuring saturation levels, and suddenly they dip or they increase, it could be an early warning signal,” he says.
“Saturation monitoring has been around for more than 30 years. But it’s the positioning of it, and the application to the different sites, that is interesting.”