The large grey domed structure sits incongruously against a crisp blue sky, ringed by dramatic mountains in the middle-distance. The air tastes Alpine-spring fresh to everyone but the locals.
Under the grey dome, a 58MW nuclear research reactor bubbles away, producing a constant supply of neutrons scientists use to look inside materials at the atomic level, to reveal information about their structure, how they move and their magnetic properties.
Unusually for a nuclear research facility, the Institut Laue-Langevin (ILL) is located within the French city of Grenoble's city limits, less than two miles from the centre. The reactor draws its cooling water from the nearby River Drac, which runs through the city.
Despite its proximity, Grenoble's inhabitants are as non-plussed by the nuclear reactor as they are the fresh air.
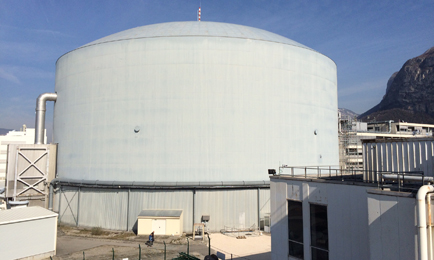
The reactor building has been substantially reinforced post-Fukushima
The ILL's reactor was built in 1971. The reactor produces neutrons that scientists harness to look inside materials at the atomic level, to reveal information about their structure, how they move and their magnetic properties.
Around 600 scientific papers a year are produced using experiments conducted at the ILL, more than at any other neutron source. A collaboration between France, the UK and Germany, the ILL's success has helped to develop one of the most impressive concentrations of science and technology research expertise in the world at Grenoble. The ILL campus also hosts the European Synchotron, which uses X-rays to analyse materials, and a branch of the European Molecular Biology Laboratory that focuses on structural biology.
Professor William Stirling, director of the ILL, says that despite its age the ILL reactor is still “the most powerful research reactor in the world”. This is impressive considering the amount of competition. There are 16 neutron sources in Europe, 10 in the US, eight in Asia, three in Oceania and one in South America. There has not been a significant increase in the power or efficiency of neutron sources since the ILL was built.
Essentially, the ILL's reactor works in the the same way as a nuclear power plant's. It is fuelled by enriched uranium 235.
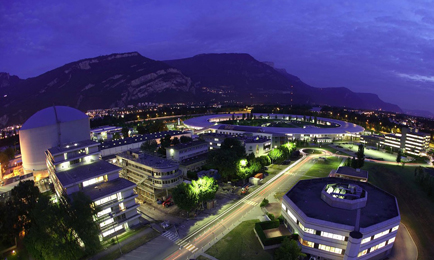
The Grenoble site also hosts the European Synchrotron
However, unlike a nuclear power plant, which uses heat to produce power, the ILL reactor's function is to produce neutrons. “The neutron production process also produces heat, which is what you want with nuclear power plants,” says Stirling. “But with research reactors you want to get the heat out of the way. The ILL is about as good as you can get, between 1-3MW per litre.”
The neutrons bounce around inside the reactor vessel at speeds of up to 20,000 km/s, and are slowed down by the heavy water that surrounds the central uranium fuel pod and cools it. This heavy water, which has a temperature of around 35°C, is heat exchanged with the light water from the river.
There are 13 neutron “beam tubes” placed at different angles into the core. The tubes, which are made from aluminium alloy and have to be replaced every 8-12 years, are designed to take in the neutrons after they have collided with the heavy water molecules and the wall of the reactor vessel. Neutrons are also reflected back into the core to sustain the nuclear reaction.
From the beam tubes the neutrons are channelled through “neutron guides”. These were pioneered at the ILL and are now commonly used at other neutron research facilities. Neutron guides enable the delivery of beams of neutrons further away from the reactor than would otherwise be possible. In the ILL this is up to 100m away, into one of 39 different scientific instruments designed and developed by teams of scientists and engineers. The ILL employs around 500 staff, many of which are engineers.

The reactor control room
The instruments are where the research happens. The diffractometers, reflectometers and spectrometers housed within the two cavernous “guide halls” next to the reactor, vary in size and complexity. Over the decades research conducted in the ILL has spanned almost every conceivable sector of science and engineering: from revealing the stress and strain fatigue in jet engine turbine blades, to improving anti-HIV drugs and identifying antifreeze proteins in Antarctic fish blood.
The instruments and experiments all run independently and simultaneously. Around a quarter of the experiments are researching magnetism, a quarter are biology and soft materials - liquids, gels and jellies. The remainder are materials science, nuclear and particle physics experiments. The ILL estimates that around 20% of the research is close-to-market materials and products, although Stirling says that the development of new materials is rarely done for purely academic reasons.
Stirling says: “The site has one of the highest concentrations of science and technology expertise in the world, and is behind numerous innovations in areas such as electronics, IT, energy and health. Almost everything we know about the behaviour of complicated magnetic materials comes from neutrons.”
Neutrons and X-rays can tell an engineer more about a material at the structural level, about its performance in applications and the residual stresses and strains within it. “It can provide information well beyond what an industrial laboratory can provide and do it in a very short time,” says Stirling.
For example, in electronics, neutron research can reveal how electrons move in a microchip. Neutrons are used to develop new polymers, fuel cells or pharmaceuticals. The range of applications is breathtaking.
Each instrument costs £3-6 million to develop. Dr Bob Cubitt, researcher at ILL, says: “Scientists determine the specification and how the machine is going to work and the engineers develop it.
“The main challenge with neutrons is that they are neutral. X-rays work with electrons, which are easy to detect. Neutrons can make gamma rays, but they are hard to detect. Most detectors use helium which the neutrons ionise. It's a technique we use every day to get readings.”
The main recent technological advancements at the ILL have included improvements to the coatings of the neutron guides, which has increased the amounts of neutrons that can be delivered to the instruments. The neutron detectors' size and effectiveness has also been improved. Charles Dewhurst, a researcher at the ILL, says: “It's a continuous process of getting bigger, better and faster - of restructuring and replacing the instruments. A new instrument can take years to design, than usually a year to build from scratch. In neutron science we get better by an order of magnitude every ten years.”
As long as the reactor is producing neutrons, it seems likely the experiments will continue. Stirling, director of the ILL, sees no technical reason why the reactor cannot continue operating “up to and even beyond the 2030's”.
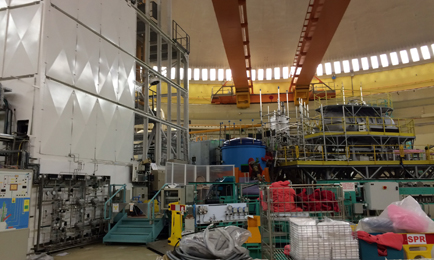
The top floor of the reactor building during an outage
However there are threats to the reactor's continued operation. The disaster at the Fukushima nuclear power plant in 2011 caused a flurry of regulatory activity and inspection at nuclear plants across the world. Governments turned to their nuclear industries and asked: could this happen here?
The ILL, with its location within Grenoble, set within a valley surrounded by mountains, has proved an early and some might say “soft” target for safety improvements. An extra £15 million had to be provided by the ILL's funding organisations for the upgrades and modifications.
“We've had to reinforce the reactor to withstand an earthquake up to Richter 7.3, which is estimated to occur once every 50,000 years” says Stirling. “The scenario we've worked to includes the breaching of four dams on the River Drac, a 10m deep flood and the release of phosgene from a chemical plant upriver.”
In the last 18 months a new emergency control room has been built on the roof of the reactor building. The room is hermetically sealed and can survive a direct helicopter crash. A 2.5m deep concrete wall has been built to protect the concrete foundations of the reactor building. Extra generators, plant and equipment has been installed to provide cooling water for the reactor. Wells have been drilled to provide a groundwater circuit of cooling water. Almost everything around and connected to the reactor building has been reinforced. A zip wire system for transport between buildings has been devised to circumvent the flood.
But there are also non-technical threats to the reactor. The UK seemingly is putting more money into spallation neutron sources after building Isis in Oxfordshire. Spallation, where neutrons are created by accelerating and then smashing particles together, is not a nuclear process, making safety and security cheaper and easier. The other founder member of the ILL Germany, has a very strong anti-nuclear stance after Fukushima.
There is also a newcomer being built. When the European Spallation Source (ESS) in Lund, Sweden, is completed in 2020, it will become the largest neutron source in the world. The ESS is costing €1.8 billion to build and will cost €200 million to run every year. However, like all spallation sources, the neutrons it produces will be in bursts, not a continuous pipeline of neutrons like the ILL.
Researcher Dr Bob Cubbitt says: “The neutron source at the ILL is on steady and that is a huge advantage. With spallation sources the beams are unpredictable. They are not reliable. It's not a technical reason more spallation sources are being developed, it's a political one.”
However, the more immediate challenge is the cost of the reactor's fuel. Currently supplied by a subsidiary of Areva, the High Flux Reactor (HFR) fuel is a niche, unusually shaped product, even within the specialist area of uranium-enriched nuclear reactor fuel. The HFR fuel element is only used by a small number of reactors in Germany, Belgium and France. The ILL is about four years into a ten year programme to convert the reactor to the more common Low Enriched Uranium fuel type.
Despite the technical and operational challenges, the value of the scientific and industrial research generated by the ILL cannot be denied. Engineers have worked hard to ensure the facility has a future post-Fukushima and the upgrades have not come cheap. It seems unlikely its future will be curtailed. Neutrons may be invisible and pass through objects with ease, but their impact has and continues to be, massive.